The more I learn about quantum technologies, the more surprised I am that quantum sensors are not widely known. I decided to do my part with this post.
Thanks to my favourite communication partner Chris for finely tuning this edition of Unbeaten Path with the Pizza Hotline.
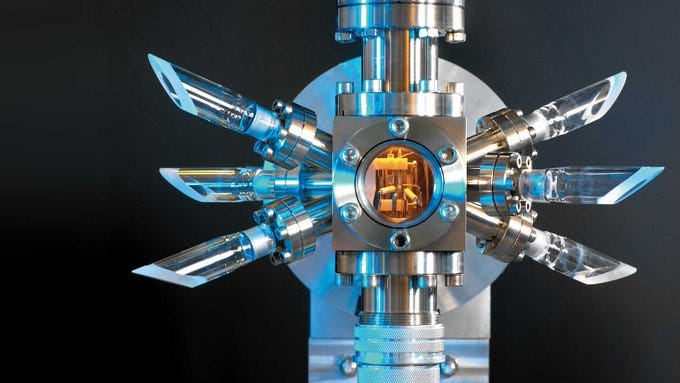
Quantum computing has long been a feature in pop culture. When Quantum Leap appeared on TV screens last autumn, I didn’t realise it was a revival of the same-titled show from the 1980s. I was born just as the original series finished in 1993.
Ever since, quantum computing has been tipped to change the world any point soon. Sticking with the American TV theme, if quantum computing is the cool kid in town, then who’s the underdog we’re all rooting for?
Who’s the unpopular geek who’s undeservingly hiding in the shadows that everyone wants to admire?
The unassuming hero in this story is quantum sensing. The ugly friend with huge potential that only needs to emerge from the shadows. Step into the spotlight, my friend.
One of the main reasons why quantum sensors go unnoticed is that the line between quantum and classical sensors is blurred.
The World is Quantum
Take your smartphone camera for example: light travels through the lens, hits the image sensors and, thanks to the photoelectric effect, generates an electrical signal. This is then rendered by the camera as an image.
Few would suggest that a phone’s camera is a quantum sensor. But guess what? The photoelectric effect is a quantum effect.
The world is quantum but, when a huge number of quantum particles are taken as a whole, they drop quantum mechanics like a bad habit. They stroll into the world of classical physics, popularised by Sir Isaac Newton.
We can use this distinction to draw a line between quantum and classical sensors. Sensors are “quantum” when they need to measure physical phenomena so small that quantum mechanics still applies. In other words, a compass excels at measuring the Earth’s magnetic field, but only a quantum sensor can measure your brain’s magnetic field.
This all begs the question: “Will we ever need to peer into the quantum world, and if yes, when?”
The truth is (d‘uh) that we already do and have been for some time!
Atomic Time Keeping
Just imagine if today’s world struggled to accurately define time. Every facet of modern life relies on time. Not just your coffee appointments or annoying late Silicon Valley scheduled Zoom calls. But the real stuff like understanding precise positions via GPS to synchronizing communications in data centers and validating financial transactions.
None of this would be possible without the most important quantum sensor around: the atomic clock.
A Swatch regulates time with the oscillation of a quartz crystal. This gem vibrates at a near-perfect frequency when electricity is applied to it. However, it’s not good enough for modern economies. Even the best ones are an entire nanosecond late after just an hour of operation.
One nanosecond seems cute, but if this error persists for just 6 weeks, using it to calculate the estimated position of a GPS would give an error of approximately 300 kilometers.
Atomic clocks, however, use the energy difference between different orbitals in atoms. This measure is, by nature, identical in every single atom of the same element that exists in the universe. It enables atomic clocks to be incredibly precise: it takes 10 million years for an atomic clock to be delayed by a second.
Atomic clocks appear futuristic, but are commonplace: GPS satellites are jam-packed with them, and the time they track is used in all the applications we have discussed above.
Quantum Squid Games
Time matters more than ever today, but historically we’ve been more chill about it. However, magnetic fields have been essential for centuries.
Long ago, humans understood that they could sail the world by measuring the Earth’s magnetic field. Yet, it was only in the late 1800s that we understood that the electrical and magnetic fields are two faces of the same coin: the electromagnetic field.
We can measure magnetic fields to understand what’s happening in systems where electricity is flowing. The coolest example of this is our brain. Neurons communicate through electrical signals and mapping these signals has told us more about the human brain than anything else.
However, there’s a caveat. Our brain’s electrical currents are very weak (you’ve never noticed them, right?) and so is the magnetic field they generate. A “classical” compass isn’t sensitive enough to record them, so again we need to rely on a quantum sensor.
The quantum sensor used here is a SQUID, or Superconducting Quantum Interface Device. It’s deployed in Magnetoencephalography, which is the neuroimaging technique used to “see” our brains in action, and Magnetic Resonance Imaging, which is a well-known radiology imaging technique for observing our inner anatomy and physiological processes. You may know it simply as an “MRI”.
SQUIDs make use of superconductors' defining property: materials that don’t oppose any resistance to the passage of currents that are weaker than a given threshold, known as “critical current”.
Our brain’s electrical activity generates a very weak magnetic field and this induces a current in the SQUID. When the current exceeds the superconductor’s critical current, a resistance appears and the resulting voltage can be measured.
SQUIDs are incredibly sensitive and allow us to learn incredible things about our bodies.
It’s Time for Sensors
Many other quantum sensors are already being used today.
From inspecting defects in semiconductors and exploiting defects in diamonds to absolute gravity measurements using laser-cooled atoms.
As Elon Musk would say, let that sink in. Quantum sensors are already being used, right here and right now. Not in 5, 10, or 15 years’ time.
It’s about time we shine some light on these amazing applications of quantum mechanics.
It’s time for sensors to take center stage.